안녕하세요, WebGL과 WebGPU를 기반으로 하는 R3F를 이용한 3D 가상환경 멀티플레이어 인터렉티브 웹 개발에 대한 교육을 소개합니다.
수강신청은 아래의 이미지를 클릭 하시면 해당 링크로 이동합니다.
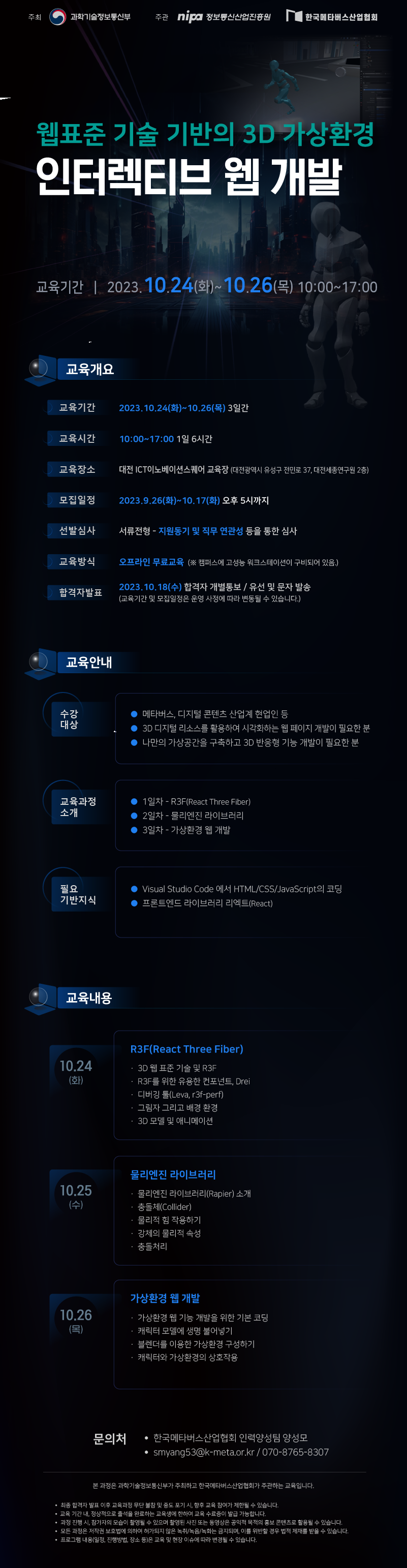
공간정보시스템 / 3차원 시각화 / 딥러닝 기반 기술 연구소 @지오서비스(GEOSERVICE)
버텍스 쉐이더에서 정점을 흔들었을 경우 법선 벡터 역시 다시 계산을 해줘야 하는데, 이때 정점의 x와 y에 대한 편미분 값을 얻을 수 있는 dFdx와 dFdy를 이용하면 법선 벡터를 얻을 수 있습니다.
이런 경우 버텍스 쉐이더에 전달된 normal을 vary를 통해 프레그먼트 쉐이더로 전달할 필요가 없고 프레그먼트 쉐이더에서 법선 벡터를 계산해 주면 되는데, 프레그먼트 쉐이더에서 이에 대한 코드는 다음과 같습니다.
vec3 normal = normalize( cross( dFdx(vPosition.xyz), dFdy(vPosition.xyz) ) );
위의 vPosition은 버텍스 쉐이이더에서 재계산된 정점인데, 버텍스 쉐이더의 코드에서 보면 다음과 같습니다.
varying vec3 vPosition; void main() { vec3 posClone = position; posClone = /* 정점 흔들기(변경) */ ... vPosition = modelMatrix * vec4(posClone, 1.0)).xyz; ... }
적용 결과로 비교하면 먼저 dFdx와 dFdy를 통한 법선 벡터를 사용하지 않고 지오메트리를 통해 제공되는 법선 벡터를 그대로 사용한 경우는 아래와 같습니다.
버텍스 쉐이더에서 정점을 흔들어서 원래 제공된 법선 벡터가 맞지 않아 음영 효과가 제대로 표현되지 않는데, 이를 개선하기 위해 dFdx와 dFdy를 통한 법선 벡터를 사용한 결과는 다음과 같습니다.